QSense耗散型石英晶體微天平在制藥領(lǐng)域中的應用
瀏覽次數(shù):807 發(fā)布日期:2024-6-13
來源:本站 僅供參考,謝絕轉(zhuǎn)載,否則責任自負
QSense耗散型石英晶體微天平在制藥領(lǐng)域中的應用
QSense 耗散型石英晶體微天平技術(shù)
耗散型石英晶體微天平(QCM-D)技術(shù)是一項表界面敏感且多功能的分析技術(shù),主要用于研究薄膜、生物分子相互作用以及其他與表界面相關(guān)的過程。QCM-D技術(shù)能夠提供關(guān)于分子在固體表面吸附、相互作用和穩(wěn)定性的寶貴見解。
QCM-D技術(shù)可以實時監(jiān)控石英晶體芯片的諧振頻率隨時間的變化。芯片的諧振頻率取決于其質(zhì)量,因此頻率的變化可以揭示與芯片表面耦合的質(zhì)量變化。同時,還可以實時監(jiān)控系統(tǒng)的能量損失(耗散),從而量化芯片上涂層的粘彈性特性。這些頻率和耗散的變化可用于分析分子與芯片表面的相互作用。
QCM-D技術(shù)是由瑞典百歐林科技有限公司和瑞典查爾姆斯理工大學的科學家們共同開創(chuàng)的,自1999年第一臺原型機問世以來,QSense耗散型石英晶體微天平產(chǎn)品系列不斷擴大。如今,QSense已成為石英晶體微天平儀器的世界領(lǐng)導者,廣泛應用于制藥、生物技術(shù)、能源、電子、材料、食品、環(huán)境、化學、礦物加工等領(lǐng)域。數(shù)千篇文獻證明了QSense技術(shù)的可靠性。以下將主要探討QSense耗散型石英晶體微天平技術(shù)在藥物相互作用方面的應用。
(一)藥物開發(fā)
QSense的納克級別質(zhì)量靈敏度為藥物發(fā)現(xiàn)和開發(fā)提供了無限潛力。通過QSense進行的研究活動包括:
- 各種實驗條件下,實時精確監(jiān)測小分子藥物與蛋白質(zhì)、細胞膜和RNA的相互作用。[1]
- 蛋白質(zhì)-蛋白質(zhì)相互作用[2]
- 小分子與RNA相互作用時,RNA的結(jié)構(gòu)變化[3]
(二)藥物遞送
QSense已被證明是一種成本效益高、時間效率高的技術(shù),特別適用于表征脂質(zhì)納米顆粒(LNP)及其藥物遞送特性方面。大量文獻證明QSense可以用于:
- 分析血清蛋白與脂質(zhì)納米顆粒(LNP)的結(jié)合親和力[4]
- 生物分子(如siRNA和mRNA)在LNP上的結(jié)合與釋放[5]
- 將LNP遞送到目標器官[6]
- 在無細胞環(huán)境中篩選血清蛋白與LNPs的結(jié)合親和力[7]
- 分析LNPs的表面修飾[8]
- 脂質(zhì)與生物活性分子(包括藥物、DNA和siRNA)的相互作用[9]
- ApoE結(jié)合后對脂質(zhì)成分分布和整體LNP結(jié)構(gòu)的影響[24]
- 用于存儲功能化LNP的納米孔陣列[25]
- 提高LNPs核酸載荷遞送效率的LNP配方[26]
- 使用cDNA將微泡固定到支持的脂質(zhì)雙層上[27]
- 穩(wěn)定化立方體的嵌段共聚物與生物模擬脂質(zhì)膜的相互作用[28]
(三)藥物-表面相互作用
QSense在表征藥物配方與表面相互作用方面具有重要意義,涵蓋了生產(chǎn)、純化、儲存和遞送過程中的多個方面。特別是評估生物制藥藥品在整個生命周期中的表面吸附/解吸附過程以及吸附層的結(jié)構(gòu)變化。
典型案例包括:
- 藥物與聚合物、玻璃、金屬和金屬氧化物、硅油等表面的相互作用[10],[11],[12],[13],[14],[15],[16]
- 輔料在減少藥物-蛋白質(zhì)吸附到表面上的效果[17]
- 配方條件(濃度、pH值、溫度等)的影響; [18]
- 界面和界面應力在生物制品開發(fā)中的影響[19]
藥物-表面相互作用研究用QCM-D芯片列表 |
塑料包裝 |
聚丙烯 (PP)
聚氯乙烯 (PVC)
聚對苯二甲酸乙二醇酯 (PET)
聚甲基丙烯酸甲酯 (PMMA) |
聚乙烯 (PE)
低密度聚乙烯 (LDPE)
高密度聚乙烯 (HDPE)
線性低密度聚乙烯 (LLDPE) |
玻璃容器 |
硼硅酸鹽玻璃 |
蘇打石灰玻璃 |
包裝袋 |
環(huán)烯烴聚合物 (COP) |
環(huán)烯烴共聚物 (COC) |
過濾材料 |
聚偏二氟乙烯 (PVDF)
聚四氟乙烯 (PTFE)
聚碳酸酯 (PC) |
聚醚砜 (PES)
聚對苯二甲酸乙二醇酯甘油改性 (PET-G) |
預充填注射器 |
注射器 PDMS(硅油) |
其他相關(guān)材料 |
聚苯乙烯
纖維素
不銹鋼L605
SS2343(類似于美國標準316)
乙烯-醋酸乙烯共聚物 (EVA) |
尼龍
聚氨酯
醋酸纖維素
聚丙烯腈 (PAN) * |
*注:多達 200 種芯片,可根據(jù)用戶要求定制芯片表面
(四)生物材料與人體組織的相互作用
植入體和生物材料在人體內(nèi)的生物相容性是它們成功發(fā)揮作用的關(guān)鍵。QSense提供了在分子層面對植入體表面或生物材料與人體血液和組織相互作用的體外分析。
- 各種眼部護理配方與黏蛋白/細胞膜表面的相互作用[20]。
(五)生物傳感器開發(fā)
QSense也被廣泛用于蛋白質(zhì)生物傳感器和即時檢測傳感器等類型傳感器的開發(fā)中。
- 蛋白質(zhì)生物傳感器[21],[22]
- 即時檢測傳感器(Point-of-care sensors)[23]
QSense Omni 耗散型石英晶體微天平
QSense Omni 是由QCM-D技術(shù)的先驅(qū)者瑞典百歐林科技有限公司推動研發(fā)的最新耗散性石英晶體微天平型號,是QCM-D最新技術(shù)的集大成者。Omni比市面上任何一款QCM的靈敏度都要高,這使它能夠量化和監(jiān)測更小的分子、更快的過程,是研究生物過程非常理想的工具。QSense有超過100多種芯片表面材料和涂層可供選擇,支持模擬真實生物環(huán)境和過程,以表征蛋白質(zhì)吸附速率、薄膜形成、吸附層剛性、鈣化、細胞附著等。
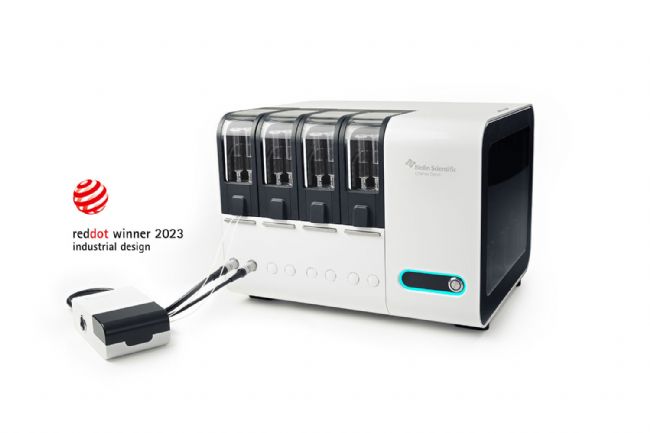
QSense Omni 耗散型石英晶體微天平
- 能夠檢測芯片表面微小至0.24 ng/cm²的變化
- 更快的流體交換(5倍于上代產(chǎn)品),提供更快和更清晰的樣品輸送
- 全系列自動化功能,最小化用戶依賴性
- 更簡化的工作流程和全新直觀的軟件界面,使更廣泛的用戶可以更加容易地使用QCM-D。
參考文獻:
[1] Small-molecule-mediated control of the anti-tumour activity and off-tumour toxicity of a supramolecular bispecific T cell engager Nat. Biomed. Eng 2024, 8 (5), 513–528. https://doi.org/10.1038/s41551-023-01147-6.
[2] Genentech – Viscoelastic characterization of high concentration antibody formulations using quartz crystal microbalance with dissipation monitoring Journal of Pharmaceutical Sciences 2009, 98 (9), 3108–3116.
https://doi.org/10.1002/jps.21610.
[3] Roche – Reconstitution and Functional Analysis of a Full-Length Hepatitis C Virus NS5B Polymerase on a Supported Lipid Bilayer ACS Cent. Sci. 2016, 2 (7), 456–466. https://doi.org/10.1021/acscentsci.6b00112.
[4] A Fast and Reliable Method Based on QCM-D Instrumentation for the Screening of Nanoparticle/Blood Interactions Biosensors 2023, 13 (6), 607. https://doi.org/10.3390/bios13060607.
[5] A QCM-D and SAXS Study of the Interaction of Functionalised Lyotropic Liquid Crystalline Lipid Nanoparticles with siRNA ChemBioChem 2017, 18 (10), 921–930. https://doi.org/10.1002/cbic.201600613.
[6] Helper lipid structure influences protein adsorption and delivery of lipid nanoparticles to spleen and liver Biomater. Sci. 2021, 9 (4), 1449–1463.
https://doi.org/10.1039/D0BM01609H.
[7] AstraZeneca – Screening of the binding affinity of serum proteins to lipid nanoparticles in a cell free environment Journal of Colloid and Interface Science 2022, 610, 766–774. https://doi.org/10.1016/j.jcis.2021.11.117.
[8] Insights into the mechanisms of interaction between inhalable lipid-polymer hybrid nanoparticles and pulmonary surfactant Journal of Colloid and Interface Science 2023, 633, 511–525.
https://doi.org/10.1016/j.jcis.2022.11.059.
[9] On the interactions between RNA and titratable lipid layers: implications for RNA delivery with lipid nanoparticles Nanoscale 2024, 16 (2), 777–794.
https://doi.org/10.1039/D3NR03308B.
[10] Genentech – Adsorption and Aggregation of Monoclonal Antibodies at Silicone Oil–Water Interfaces Mol. Pharmaceutics 2021, 18 (4), 1656–1665. https://doi.org/10.1021/acs.molpharmaceut.0c01113.
[11] Bristol-Myers Squibb – Mechanistic Understanding of Protein-Silicone Oil Interactions Pharm Res 2012, 29 (6), 1689–1697.
https://doi.org/10.1007/s11095-012-0696-6.
[12] Bristol-Myers Squibb – Adsorption of polypropylene oxide-polyethylene oxide type surfactants at surfaces of pharmaceutical relevant materials: effect of surface energetics and surfactant structures Pharmaceutical Development and Technology 2019, 24 (1), 70–79. https://doi.org/10.1080/10837450.2018.1425431.
[13] Bristol-Myers Squibb – Particle Characterization for a Protein Drug Product Stored in Pre-Filled Syringes Using Micro-Flow Imaging, Archimedes, and Quartz Crystal Microbalance with Dissipation AAPS J 2017, 19 (1), 110–116.
https://doi.org/10.1208/s12248-016-9983-1.
[14] Pfizer – Engineering a ceramic piston pump to minimize particle formation for a therapeutic immunoglobulin: A combined factorial and modeling approach. J Adv Manuf & Process 2023, 5 (1), e10142.
https://doi.org/10.1002/amp2.10142.
[15] Antibody adsorption and orientation on hydrophobic surfaces Langmuir 2012, 28 (3), 1765–1774.
https://doi.org/10.1021/la203095p.
[16] AstraZeneca – The Impact of the Metal Interface on the Stability and Quality of a Therapeutic Fusion Protein Mol. Pharmaceutics 2020, 17 (2), 569–578. https://doi.org/10.1021/acs.molpharmaceut.9b01000.
[17] Janssen Pharmaceuticals (Johnson and Johnson) – Quartz Crystal Microbalance as a Predictive Tool for Drug-Material of Construction Interactions in Intravenous Protein Drug Administration Journal of Pharmaceutical Sciences 2023, 112 (12), 3154–3163. https://doi.org/10.1016/j.xphs.2023.07.019.
[18] Eli Lilly – Surface Interactions of Monoclonal Antibodies Characterized by Quartz Crystal Microbalance with Dissipation: Impact of Hydrophobicity and Protein Self-Interactions Journal of Pharmaceutical Sciences 2012, 101 (2), 519–529.
https://doi.org/10.1002/jps.22771.
[19] Bristol-Myers Squibb – Overview of the Impact of Protein Interfacial Instability on the Development of Biologic Products In Protein Instability at Interfaces During Drug Product Development; Li, J., Krause, M. E., Tu, R., Eds.; AAPS Advances in the Pharmaceutical Sciences Series; 2021; Vol. 43, pp 1–8.
https://doi.org/10.1007/978-3-030-57177-1_1.
[20] Novartis Pharma – Understanding the adsorption and potential tear film stability properties of recombinant human lubricin and bovine submaxillary mucins in an in vitro tear film model Colloids and Surfaces B: Biointerfaces 2020, 195, 111257. https://doi.org/10.1016/j.colsurfb.2020.111257.
[21] Dual-mode and Label-free Detection of Exosomes from Plasma Using an Electrochemical Quartz Crystal Microbalance with Dissipation Monitoring Anal. Chem. 2022, 94 (5), 2465–2475. https://doi.org/10.1021/acs.analchem.1c04282.
[22] Amplified QCM-D biosensor for protein based on aptamer-functionalized gold nanoparticles Biosensors and Bioelectronics 2010, 26 (2), 575–579. https://doi.org/10.1016/j.bios.2010.07.034.
[23] Bioactivated PDMS microchannel evaluated as sensor for human CD4+ cells – The concept of a point-of-care method for HIV monitoring. Sensors and Actuators B: Chemical 2007, 123 (2), 847–855.
https://doi.org/10.1016/j.snb.2006.10.034.
[24] Apolipoprotein E Binding Drives Structural and Compositional Rearrangement of mRNA-Containing Lipid Nanoparticles. ACS Nano 2021, 15 (4), 6709–6722. https://doi.org/10.1021/acsnano.0c10064.
[25] Development of Nanopackaging for Storage and Transport of Loaded Lipid Nanoparticles. . Nano Lett. 2023, 23 (14), 6760–6767.
https://doi.org/10.1021/acs.nanolett.3c01271.
[26] Review of structural design guiding the development of lipid nanoparticles for nucleic acid delivery. Current Opinion in Colloid & Interface Science 2023, 66, 101705. https://doi.org/10.1016/j.cocis.2023.101705.
[27] QCM-D Investigations on Cholesterol–DNA Tethering of Liposomes to Microbubbles for Therapy. J. Phys. Chem. B 2023, 127 (11), 2466–2474.
https://doi.org/10.1021/acs.jpcb.2c07256.
[28] Thermo-responsive lipophilic NIPAM-based block copolymers as stabilizers for lipid-based cubic nanoparticles. Colloids and Surfaces B: Biointerfaces 2022, 220, 112884. https://doi.org/10.1016/j.colsurfb.2022.112884.